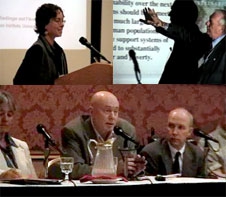
Overview
In addition to highlighting important arctic research through the Arctic Forum, the ARCUS annual meeting serves to gather member institution representatives, the Board of Directors, and the staff of ARCUS together with other members of the arctic research community and key agency personnel and policy makers.
The Arctic Forum 2002 was chaired by Dr. Sue Moore, of the National Oceanic and Atmospheric Administration National Marine Mammal Laboratory, and included invited and contributed talks by arctic researchers representing diverse disciplines, projects, and programs. This Arctic Forum focused on biocomplexity in the Arctic. Dr. Rita Colwell, Director of the National Science Foundation, gave the keynote presentation on 16 May 2002. The student recipients of the Sixth Annual ARCUS Award for Arctic Research Excellence presented their award-winning papers during the Forum as well.
The Annual Reception included posters on arctic research.
2002 Arctic Forum Volume
The volume of abstracts from the 2002 Arctic Forum are available below in PDF format.
List of Abstracts
Display: Association of Canadian Universities for Northern Studies
Frances Abele1
1President, Association of Canadian Universities for Northern Studies, 17 York St., Suite 405, Ottawa, ON, K1N 9J6, Canada, Phone (613) 562-0515, Fax (613) 562-0533, acuns [at] cyberus.ca
The Association of Canadian Universities for Northern Studies (ACUNS) represents Canada’s northern and polar researchers working at member universities and colleges. Founded in 1977, in Churchill, Manitoba, as a non-profit organization, the Association has six important functions: 1.) to represent interests of members by promoting policies and practices that support northern scholarship, 2.) to establish mechanisms through which resources can be allocated to members so as to increase knowledge of the north and ensure northern training, 3.) to enhance opportunities for northern people to become leaders and promoters of excellence in education and research important to the north, 4.) to facilitate the understanding and resolutions of northern issues, 5.) to initiate programs which will increase public awareness of northern sciences and research, 6.) to co-operate with other organizations, public, private, and international, concerned with northern studies.
Rapid Wastage of Alaska Glaciers and their Contribution to Rising Sea Level (Previously entitled: Elevation and volume changes of Alaskan glaciers, measured from airborne laser altimetry)
Anthony Arendt1, K. Echelmeyer2, W. Harrison3, C. Lingle4, V. Valentine5
1Department of Geophysics, University of Alaska Fairbanks, PO Box 757320, Fairbanks, AK, 99775-7320, USA, Phone 907-474-7146, Fax 907-474-7290
2Department of Geology and Geophysics, University of Alaska Fairbanks, PO Box 755780, Fairbanks, AK, 99775-5780, USA, Phone 907/474-7477, Fax 907/474-7290, kechel [at] gi.alaska.edu
3Geophysical Institute, University of Alaska Fairbanks, Fairbanks, AK, 99775-7320, USA
4Geophysical Institute, University of Alaska Fairbanks, Fairbanks, AK, 99775-7320, USA
5University of Alaska Fairbanks, PO Box 757320, Fairbanks, AK, 99775-7320, USA, Phone 907-474-7146, Fax 907-474-7290
We have used airborne laser altimetry to estimate volume changes of 67 glaciers in Alaska from the mid-1950s to the mid-1990s. The average rate of thickness change of these glaciers was -0.45 m/year. Extrapolation to all glaciers in Alaska yields an estimated total annual volume change of -52±7 km3/year (water equivalent), equivalent to a rise in sea level (SLE) of 0.14±0.02 mm/year. Repeat measurements of 27 glaciers from the mid-1990s to 2000-01 suggest an increased average rate of thinning, -1.1 m/year. This leads to an extrapolated annual volume loss from Alaska glaciers equal to -91±28 km3/year, or 0.25±0.08 mm/year SLE, during the last decade. These recent losses are about 78% larger than the estimated annual loss from the entire Greenland Ice Sheet during the same time period, and are much higher than previously published loss-estimates for Alaska glaciers. They form the largest glaciological contri-bution to rising sea level yet measured.
Sea Surface Temperature Fronts of the Arctic and Subarctic Seas From Satellite Data
Igor M. Belkin1
1Graduate School of Oceanography, University of Rhode Island, 215 South Ferry Road, Narragansett, RI, 02882, USA, Phone 401-874-6533, Fax 401-874-6728, ibelkin [at] gso.uri.edu
The Arctic/Subarctic Seas feature a variety of fronts that clearly manifest in the surface layer, both in temperature and salinity. Thermal fronts were studied from the Pathfinder satellite SST fields, 1985-1996, obtained from the AVHRR 9-km twice-daily images (8,364 images in total). The SST fronts were detected from each individual image using the Cayula-Cornillon front detection and declouding algorithms. Long-term (1985-1996) frontal frequencies (normalized on cloudiness) were computed for each 9-km pixel and mapped for the Arctic Ocean marginal seas and the northern North Atlantic subarctic seas. Analysis of synoptic frontal SST maps together with frontal frequency maps allowed us to distinguish a number of new fronts and elucidate important features of some previously known fronts, especially with regard to their spatial structure and its seasonal and interannual variability.
The Arctic Ocean marginal fronts are best developed in the Chukchi and Beaufort Seas in summer when the Chukchi Sea and southern Beaufort Sea become ice-free. Most fronts are topographically controlled by shelf break (e.g. in the southern Beaufort Sea), canyons (Herald Canyon, Barrow Canyon) and shelf banks (e.g. Herald Shoal and Hanna Shoal). In both seas the thermal fronts are spatially associated with distribution of biota, including sea birds and marine mammals.
Major fronts of the Nordic Seas are topographically controlled. The East Greenland Front, West Spitsbergen Front and Norwegian Coastal Front are shelf-slope fronts aligned with the respective shelf breaks/upper slopes; the Norwegian Atlantic Front is controlled by the Voring Plateau's western flank; the Iceland-Faroe Front extends over the Iceland-Faroe Ridge, while the Jan Mayen Front is located over the Jan Mayen Ridge and Mohn Ridge. The Iceland Sea frontal pattern is more complex than previously known and displays a "horseshoe" pattern formed by the East Icelandic and Kolbeinsey Ridge fronts. In the Labrador Sea, the West Greenland Front follows the shelfbreak. The Baffin Front emerges as an independent feature, originated largely in the Baffin Bay. The offshore Labrador Front is fairly stable, aligned with the shelfbreak. In summer, the newly identified mid-shelf Labrador Front is also observed. This double-front structure sometimes persists through October.
This study was funded by the NASA (grant No. 535834), whose support is gratefully acknowledged.
Subsurface Warming Versus Surface Cooling of the North Pacific Polar Front
Igor M. Belkin1, Richard Krishfield2, Susumu Honjo3
1Graduate School of Oceanography, University of Rhode Island, 215 South Ferry Road, Narragansett, RI, 02882, USA, Phone 401-874-6533, Fax 401-874-6728, ibelkin [at] gso.uri.edu
2Department of Geology and Geophysics, Woods Hole Oceanographic Institution, Woods Hole, MA, 02543, USA, Phone 508-289-2849, rkrishfield [at] whoi.edu
3Joint North Pacific Research Center, Woods Hole Oceanographic Institution, Woods Hole, MA, 02543, USA, Phone 508-457-2000, shonjo [at] whoi.edu
Over 200 meridional sections in the northern North Pacific are analyzed, augmented with a number of surveys in the Gulf of Alaska, in order to map the Polar Front and study its long-term variability (Belkin et al., 2002). The Polar Front is defined as the southern boundary of the “pure” Subarctic vertical structure that features a pronounced, very cold, subsurface temperature minimum (Tmin) underlain by a temperature maximum (Tmax). This boundary is typically associated with enhanced horizontal gradients of most oceanographic parameters. These elevated gradients can be traced down to at least 1500-2000 m depth. The first trans-Pacific map of the Polar Front reveals this important physical and biogeographical boundary extending non-zonally from 40°N off Japan to 57°N in the Gulf of Alaska, where the front retroflects and continues WSW with the Alaskan Stream. The Polar Front parameters change downstream and with time. The decadal variability of the front is studied along the 150°E, 170°E, 175.5°E, and 180°E sections, occupied annually. Most time series of the Tmin, Tmax, and T100 temperatures on both sides of the front demonstrate a dramatic long-term subsurface warming between 1978 and 1999. The magnitude of the Tmin warming is at least 1°C over 20 years (up to 2°C along 170°E). Since the Tmin is a remnant of wintertime convection, this warming signals a significant amelioration of the winter climate of the northern North Pacific over the last two decades. At the same time, the summertime SST time series along the Polar Front show a concurrent cooling, about 1°C to 2°C over 20 years. The observed subsurface warming/surface cooling of the Polar Front and associated current are supposed to have been advected by the large-scale circulation to the Gulf of Alaska, Alaskan Stream and eventually, with the Alaskan Stream's northward branches, to the Bering Sea.
References:
Belkin, I.M., R. Krishfield, and S. Honjo (2002) Decadal variability of the North Pacific Polar Front: Subsurface warming versus surface cooling, Geophysical Research Letters, vol.29, in press.
Adaptations of Euro-Canadian Schools to Inuit Culture in Selected Communities in Nunavut
Paul Berger1
1Faculty of Education, Lakehead University, 955 Oliver Road, Thunder Bay, ON, P7B 5E1, Canada, Phone 807-983-2333, pberger [at] mail.lakeheadu.ca
This poster highlights aspects of a study which explored current and desired adaptations of Euro-Canadian schools to Inuit culture in five communities in one region of Nunavut, Canada.
To increase the success or well-being of Inuit students, the main recommendations from this study were: 1) to increase community ownership of schools through meaningful consultation, 2) to increase the number of Inuit educators in schools, and to support them in remaining Inuit rather than adopting Euro-Canadian ways of being/teaching, 3) to base the hiring of teachers for Northern schools on their orientation towards change and their ability to work with people, rather than on their academic qualifications, 4) to create an orientation to Inuit culture, learning styles, and communication patterns for new teachers hired from the South, and to inservice teachers on cultural and ESL issues and strategies, 5) to create and effectively distribute relevant, culturally sensitive curricula and resources in Inuktitut and (ESL sensitive) English, and, 6) to create policy which encourages teachers to prioritize the meeting of students' needs.
The poster will include points from the review of literature dealing with dominant-culture schools in minority-culture settings, a brief description of the methodology employed in the study, selected participant quotes, and a summary of the findings and recommendations.
Adaptations of Euro-Canadian Schools to Inuit Culture in Selected Communities in Nunavut
Paul Berger1
1Faculty of Education, Lakehead University, 955 Oliver Road, Thunder Bay, ON, P7B 5E1, Canada, Phone 807-983-2333, pberger [at] mail.lakeheadu.ca
This paper describes a study which explored educators perceptions of current and desired adaptations of Euro-Canadian schools to Inuit culture in five communities in one region of Nunavut. Participants in the study reported very few instances where community input was solicited, noted as desired, or used in determining the direction of the schools, and few instances where schools explicitly taught Inuit values. Many examples were given of incorporating Inuit curricula into schools, and many practices were documented which were educators attempts to structure classroom interaction in ways that mirror the cultural expectations of Inuit students. Many changes were also reported which are in fact current Southern practices, teaching ESL methods, or strategies designed to respond to the effects on students of societal problems. These changes may have increased school effectiveness, but did not move them toward Inuit culture.
To increase the success or well-being of Inuit students, the main recommendations from this study were: 1) to increase community ownership of schools through meaningful consultation, 2) to increase the number of Inuit educators in schools, and to support them in remaining Inuit rather than adopting Euro-Canadian ways of being/teaching, 3) to base the hiring of teachers for Northern schools on their orientation towards change and their ability to work with people, rather than on their academic qualifications, 4) to create an orientation to Inuit culture, learning styles, and communication patterns for new teachers hired from the South, and to inservice teachers on cultural and ESL issues and strategies, 5) to create and effectively distribute relevant, culturally sensitive curricula and resources in Inuktitut and (ESL sensitive) English, and, 6) to create policy which encourages teachers to prioritize the meeting of students' needs.
Arctic People in Complex Adaptive Systems: The Case of Climate Change
Fikret Berkes1
1Natural Resources Institute, University of Manitoba, 70 Dysart Road, Winnipeg, MB, R3T 2N2, Canada, Phone 204-474-6731, Fax 204-261-0038, berkes [at] cc.umanitoba.ca
I am going to talk about linking social systems and natural systems as complex systems, and give some examples from the area of climate change. Many of our environmental problems, including climate change, are complex systems problems, and are not adequately addressed by the familiar scientific approach of developing and testing hypotheses serially. As well, conventional disciplines are inadequate to deal with problems involving the interaction of humans with their environment. These coupled social and ecological systems (social-ecological systems for short) need to be understood and approached as complex adaptive systems.
What does complex systems theory say about social-ecological systems? I am going to concentrate on lessons from two attributes: scale and emergence. Scale really does matter; reductionist approaches have limited explanatory power because reality has a hierarchical structure. Each level along the hierarchical scale is independent, to some degree, of the levels above and below. Thus, each level requires new concepts and principles. Social-ecological systems can be studied at various levels, with some similarities and some key differences. The human component must be understood in all its social complexity, and not treated simply as a blackbox that produces feedbacks.
In the language of complexity, emergence refers to systems properties that cannot be predicted or understood simply by examining the parts of the system. Emergent properties provide a window for the study of system-wide phenomena. In our work, we have been using the resilience (buffering or absorptive capacity in the face of perturbations), for the study of change. Resilience is particularly suitable for the study of change because it deals with the flexibility of responses to stress, and it focuses on system's capacity for learning, self-organization and adaptation at multiple scales.
While working on the Epilogue of the new ARCUS book, The Earth Is Faster Now, I had a chance to think about the implications of complexity thinking for climate change and impacts in the Arctic. Cross-scale interactions are occurring both horizontally (geographically) and vertically across institutional levels (community, regional, national, international). No single level is the "correct" one for analysis; levels must be analyzed both separately and simultaneously across scale. The emergent property, resilience, helps focus on the adaptive capacity of communities to deal with climate change. Switching species and adjusting the "where, when and how" of hunting are strategies for dealing with change - up to a limit. Evolving co-management institutions create additional linkages for cross-scale feedback and help increase resilience.
Are Goose Nesting Success and Lemming Cycles Linked? Interplay Between Nest Density and Predators
Joël Bêty1, Gilles Gauthier2, Jean-Francois Giroux3, Erkki Korpimäki4
1Biology, Centre D'Études Nordiques (Northern Studies Centre), Laval University, Quebec, QC, G1K 7P4, Canada, jbety [at] birdlover.com
2Biology, Centre d'Études Nordiques, Laval University, Quebec, QC, G1K 7P4, Canada
3Sciences biologiques, Université du Québec à Montréal, Montréal, QC, Canada
4Biology, Section of Ecology, University of Turku, Turku, Finland
The suggested link between lemming cycles and reproductive success of arctic birds is caused by potential effects of varying predation pressure (the Alternative Prey Hypothesis, APH) and protective association with birds of prey (the Nesting Association Hypothesis, NAH). We used data collected over two complete lemming cycles to investigate how fluctuations in lemming density were associated with nesting success of greater snow geese (Anser caerulescens atlanticus) in the Canadian High Arctic. We tested predictions of the APH and NAH for geese breeding at low and high densities. Goose nesting success varied from 22% to 91% between years and the main egg predator was the arctic fox (Alopex lagopus). Nesting associations with snowy owls (Nyctea scandiaca) were observed but only during peak lemming years for geese nesting at low density. Goose nesting success declined as distance from owls increased and reached a plateau at 550 m. Artificial nest experiments indicated that owls can exclude predators from the vicinity of their nests and thus reduce goose egg predation rate. Annual nest failure rate was negatively associated with rodent abundance and was generally highest in low lemming years. This relationship was present even after excluding goose nests under the protective influence of owls. However, nest failure was inversely density-dependent at high breeding density. Thus, annual variations in nest density influenced the synchrony between lemming cycles and oscillations in nesting success. Our results suggest that APH is the main mechanism linking lemming cycles and goose nesting success and that nesting associations during peak lemming years (NAH) can enhance this positive link at the local level. The study also shows that breeding strategies used by birds (the alternative prey) could affect the synchrony between oscillations in avian reproductive success and rodent cycles.
Using permafrost DNA to examine the effects of environmental change
Alan Cooper1
1Department of Zoology, Oxford University, South Parks Rd, Oxford, OX26UE, UK, Phone 44-1865-271263, Fax 44-1865-271249, alan.cooper [at] zoo.ox.ac.uk
The Arctic contains many detailed paleoenvironmental records, with important implications for the interpretation of current changes in climate, biodiversity, and human impact. Recent molecular studies have revealed a further record – an Ice Age genetic museum preserved in vast numbers of mammal bones from permafrost deposits. These remains provide an opportunity to examine the genetic effects of climate change throughout the last glacial period (>60-10 Kyr), human invasion of the New World (ca. 13 Kyr), and the megafaunal mass extinction (ca. 11 Kyr) – examples of three major evolutionary phenomena seen throughout the fossil record.
Studies of permafrost DNA from several megafaunal groups (brown bears, bison, American lions) have revealed extremely dynamic population histories – characterised by localised extinctions, rapid replacements, and evidence of a strongly mosaic environment. Climate change appears to have the most significant effect on bear biodiversity, but is mediated through secondary interactions with other environmental factors. By comparing genetic data from a range of species, it should be possible to reconstruct a detailed paleoenvironmental picture for Beringia over the past 60,000 years.
This combination of paleo-genetic, -morphological, -climatic, and -ecological data provides a powerful means to examine the effects of past environmental events, and predict the impact of current changes.
This presentation is based on: Barnes, I., P. Matheus, B. Shapiro, D. Jensen, A. Cooper. (2002) Dynamics of Pleistcene Population Extinctions in Beringian Brown Bears. Science 295:2267-2270. More information can also be found at http://evolve.zoo.ox.ac.uk/.
The North Water: An Arctic Ecosystem Poised for Change
Jody W. Deming1
1School of Oceanography and Astrobiology Program, University of Washington, Box 357940, Seattle, WA, 98195, USA, Phone 206-543-0845, Fax 206-543-0275, jdeming [at] u.washington.edu
A decade of intensive research under the auspices of the International Arctic Polynya Program (IAPP) has resulted in large and very successful interdisciplinary studies of two major Arctic polynyas: the Northeast Water, which forms off the northeast coast of Greenland (77–81°N; 1991–1993 field program); and the North Water, which forms between Greenland and Canada's Ellesmere Island (76–79°N; 1997–1999 field program). The rich comparative database developed in the process has enabled recognition of key physical, biological, and biogeochemical features that determine whether a polynya ecosystem is rich or poor in overall productivity – able or not to support top predators, including human populations. The International North Water Polynya Study (NOW) in particular has revealed clear forcing factors and input terms (climatic and oceanic) required to generate and sustain a high-magnitude phytoplankton bloom over an unprecedented period (at these latitudes) of nearly six months (April to October). The tight coupling between this bloom and the higher trophic levels of the region, enabled by the precocious recruitment of zooplankton (essential prey for fish, birds, mammals), contributes to the North Water being arguably the most productive ecosystem in the Arctic and rivaling other regions of the global ocean.
These and other discoveries have caused the IAPP to develop a new approach to polynya research for the future: dedicated time-series studies on the decadal scale that can recognize and provide a solid basis for predicting the sources and effects of environmental change. Ideally, such work would go forward in multiple Arctic polynyas simultaneously; practically, the effort is proposed to begin in the North Water (to continue that begun through NOW). There, the contemporary signals – physical, biological and biogeochemical – are as strong and unambiguous as can be expected in a complex natural system; some of them appear poised for change. For example, the seasonal duration of the ice bridge (across Nares Strait), largely responsible for polynya formation (and considered responsive to hemispheric forcing), has been decreasing over the last decade. The moving polar front in the Arctic Ocean may also conceivably (and soon) alter the chemical properties (balance of silicate and nitrate) of the inflow waters to the polynya. A remarkable diversity of phytoplankton awaits the shift away from diatoms, should silicate become limiting; the response of higher trophic levels to an altered food base then emerges as an issue of prime concern. In promoting a new program of long-term time-series research on Polynyas in the Arctic's Changing Environment (PACE), the IAPP aims to help the scientific community and society at large to learn and gain from what these archetypal ecosystems of the Arctic have to teach us about our changing Earth and its resources.
The Arctic Nearshore Environment: A System Defined by Complex Hydrological and Biogeochemical Linkages and Feedbacks
Kenneth H. Dunton1
1Marine Science Institute, The University of Texas at Austin, 750 Channel View Drive, Port Aransas, TX, 78373, USA, Phone 361-749-6744, Fax 361-749-6917, dunton [at] utmsi.utexas.edu
The nearshore zone of the Arctic Ocean represents a dynamic and physical boundary between arctic coastal plain watersheds and the arctic shelf. The exchange of water, nutrients, and organic materials at the coastal boundary has a distinct effect on the productivity and structure of the nearshore shelf ecosystem. The lateral exchanges of carbon, nutrients, and other materials are largely controlled by distinct physical processes that include coastal erosion, the timing and magnitude of river discharge, sea ice distribution, ice-retreat and break-up, and wind direction. The role of these physical events in regulating biogeochemical processes has often been underestimated. For example, recent studies have concluded that contributions of sediment through coastal erosion may equal (Laptev Sea) or exceed riverine input by sevenfold (Alaska Beaufort Sea). The biogeochemical transformation and ultimate fate of this material during its transfer from terrestrial watersheds to the nearshore zone is not well understood. However, the huge influx allocthonous matter to the nearshore zone provides a focus for the study of nearshore food chains and the efficiency in which C, N, and other constituents are incorporated into consumer organisms. The diversity of marine and terrestrial organisms in nearshore communities are largely defined by the biogeochemical linkages between marine and terrestrial ecosystems, which vary considerably over both spatial and temporal scales. The role of these processes and the hydrological feedbacks between shelf waters and the adjacent terrestrial watersheds is important to our understanding of this complex and highly physical interface between land and ocean. Such knowledge is critical to our ability to predict the impacts of environmental change to indigenous populations of the Arctic Region.
Paleogenetic Assessment of Human Migration and Population Replacement in North American Arctic Prehistory
M. Geoffrey Hayes1, J.B. Coltrain2, D.H. O'Rourke3
1Department of Anthropology, University of Utah, 270 South 1400 East - Room 102, Salt Lake City, UT, 84112, USA, Phone 801/581-6251, Fax 801/581-6252, geoff.hayes [at] anthro.utah.edu
2Department of Anthropology, University of Utah, 270 South 1400 East - Room 102, Salt Lake City, UT, 84112, USA, Phone 801/585-6444, Fax 801/581-6252, coltrain [at] anthro.utah.edu
3Department of Anthropology, University of Utah, 270 South 1400 East - Room 102, Salt Lake City, UT, 84112, USA, Phone 801/581-7454, Fax 801/581-6252, dennis.orourke [at] anthro.utah.edu
Common explanations for transitions in the archaeological record include cultural diffusion of new technologies, or rapid replacement of a resident population by more recent migrants. The former involves genetic continuity across the transition, whereas the latter suggests a genetic replacement across the transition. Two interesting cases from the North American Arctic allow for an examination of these polar explanations for such transitions. Aleut prehistory is characterized as archaeologically continuous for at least the past 4000 years, but with an associated skeletal transition from dolichocranic to brachycranic occurring approximately 1000 years before present. In the eastern Canadian Arctic an early culture complex (the Dorset) was rapidly replaced by a strikingly different culture complex (the Thule) approximately 1000 BP. Our studies compare the genetic relationship across these transitions by examining DNA extracted from archaeologically recovered pre- and post-transition individuals. To date DNA has been extracted and mtDNA amplified from >35 individuals from the Aleutians and >40 individuals from the Hudson’s Bay region. A comparison of mtDNA haplogroup frequencies, defined by the presence or absence of minimally four to six restriction sites or length polymorphisms, suggests continuity in the Aleutians, since there are no significant differences between pre and post-transition populations (both approximately 70% haplogroup D, 30% haplogroup A). In the Eastern Canadian Arctic replacement is best supported since the earlier Dorset population is fixed for haplogroup D, while the later Thule population is fixed for haplogroup A.
Perspectives from the Arctic
Orville H. Huntington1
1Department of Subsistence, U.S. Fish and Wildlife Service, Vice-Chair, Alaska Native Science Commission, P.O. Box 107, Huslia, AK, 99746, USA, Phone 907/829-2266, Fax 907/829-2224, orville_huntington [at] fws.gov
The Alaska Native residents of the Arctic I have lived with everyday for the last 46 years play an important role in understanding and sensibly managing earths few resources. Past and present Traditional Ecological Knowledge of Alaska Native Tribes provide scientists with an all encompassing view of many environmental changes, through interactive observation and study which recognize Mother earths sensitivity and the place it evolves towards. Arctic scientific research complements Traditional Native Ecological Knowledge and addresses our concerns about maintaining the quality of subsistence land, water, plants, air, fish and animals, and all other elements of the environment. Contaminants continue to be transferred by air currents, water cycles and fish and wildlife migration to our region of the Arctic, through human activity that have changed the quality of air, water, land, and fish and wildlife. Complex life cycles in the Arctic surround me everyday, and everyone in the Koyukuk River ecosystem is impacted by changes in the global environment.
New Book: The Earth is Faster Now: Indigenous Observations of Arctic Environmental Change
Igor Krupnik1, Dyanna Jolly2
1Arctic Studies Center, Smithsonian Institution, 10th and Constitution Avenue NW, Washington, DC, 20560, USA, Phone 202/357-4742, Fax 202/357-2684, krupnik.igor [at] nmnh.si.edu
2Centre for Maori and Indigenous Planning and Development, Lincoln University, PO Box 84, Canterburg, 8021, New Zealand, Phone +64-3/325-2811, Fax +64-3/325-3817, dyjolly [at] ihug.co.nz
A new book published by ARCUS, in cooperation with the Arctic Studies Center, Smithsonian Institution, addresses indigenous observations of arctic environmental change and the implications of such change for arctic peoples. Despite all the attention currently being given to climate change globally and in the Arctic, indigenous perspectives are all too frequently overlooked. The Earth is Faster Now demonstrates that arctic residents have a great deal to say. Climate change simply cannot be understood and addressed without incorporating their specific and detailed views. The processes by which this can be done, however, take considerable time and effort on the part of both researchers and arctic residents.
The nine papers collected in this volume focus primarily on documenting and understanding the nature of changes that are being seen by northern indigenous residents. The special emphasis of the papers is not simply on change but also on the ways arctic peoples perceive, influence, and are influenced by their surroundings.
World Ocean Database 2001
Sydney Levitus1, Margarita Conkright2, Todd O'Brien3, Tim Boyer4, Cathy Stephens5, Ricardo Locarnini6, Hernan Garcia7, Paulette Murphy8, Daphne Johnson9, Olga Baranova10, John Antonov11, Renee Tatusko12, Robert Gelfeld13, Igor Smolyar14
1National Oceanographic Data Center-E/OC5, National Oceanic and Atmospheric Administration, 1315 East-West Highway, Silver Spring, MD, 20910, USA, Phone 301-713-3294, Fax 301-713-3303, slevitus [at] nodc.noaa.gov
2National Oceanographic Data Center-E/OC5, National Oceanic and Atmospheric Administration, 1315 East-West Highway, Silver Spring, MD, 20910, USA, Phone 301-713-3294, Fax 301-713-3303, margarita.gregg [at] noaa.gov
3National Oceanographic Data Center-E/OC5, National Oceanic and Atmospheric Administration, 1315 East-West Highway, Silver Spring, MD, 20910, USA, Phone 301-713-3294, Fax 301-713-3303, todd.o%27brien [at] noaa.gov
4National Oceanographic Data Center-E/OC5, National Oceanic and Atmospheric Administration, 1315 East-West Highway, Silver Spring, MD, 20910, USA, Phone 301-713-3294, Fax 301-713-3303, tim.boyer [at] noaa.gov
5National Oceanographic Data Center-E/OC5, National Oceanic and Atmospheric Administration, 1315 East-West Highway, Silver Spring, MD, 20910, USA, Phone 301-713-3294, Fax 301-713-3303, cathy.stephens [at] noaa.gov
6National Oceanographic Data Center-E/OC5, National Oceanic and Atmospheric Administration, 1315 East-West Highway, Silver Spring, MD, 20910, USA, Phone 301-713-3294, Fax 301-713-3303, ricardo.locarnini [at] noaa.gov
7National Oceanographic Data Center-E/OC5, National Oceanic and Atmospheric Administration, 1315 East-West Highway, Silver Spring, MD, 20910, USA, Phone 301-713-3294, Fax 301-713-3303, hernan.garcia [at] noaa.gov
8National Oceanographic Data Center-E/OC5, National Oceanic and Atmospheric Administration, 1315 East-West Highway, Silver Spring, MD, 20910, USA, Phone 301-713-3294, Fax 301-713-3303, paulette.murphy [at] noaa.gov
9National Oceanographic Data Center-E/OC5, National Oceanic and Atmospheric Administration, 1315 East-West Highway, Silver Spring, MD, 20910, USA, Phone 301-713-3294, Fax 301-713-3303, daphne.johnson [at] noaa.gov
10National Oceanographic Data Center-E/OC5, National Oceanic and Atmospheric Administration, 1315 East-West Highway, Silver Spring, MD, 20910, USA, Phone 301-713-3294, Fax 301-713-3303, olga.baranova [at] noaa.gov
11National Oceanographic Data Center-E/OC5, National Oceanic and Atmospheric Administration, 1315 East-West Highway, Silver Spring, MD, 20910, USA, Phone 301-713-3294, Fax 301-713-3303, john.antonov [at] noaa.gov
12NODC/Ocean Climate Laboratory-E/OC5, NOAA/NESDIS, 1315 East-West Highway, Room 4147, Silver Spring, MD, 20910, USA, Phone 301-713-3295, Fax 301-713-3303, renee.tatusko [at] noaa.gov
13National Oceanographic Data Center-E/OC5, National Oceanic and Atmospheric Administration, 1315 East-West Highway, Silver Spring, MD, 20910, USA, Phone 301-713-3294, Fax 301-713-3303, robert.gelfeld [at] noaa.gov
14National Oceanographic Data Center-E/OC5, National Oceanic and Atmospheric Administration, 1315 East-West Highway, Silver Spring, MD, 20910, USA, Phone 301-713-3290, Fax 301-713-3303, igor.smolyar [at] noaa.gov
In 1982, Sydney Levitus of NOAA’s Geophysical Fluid Dynamics Laboratory (GFDL) published the first global and objectively analyzed fields of temperature, salinity, and oxygen based on all data available from the NOAA National Oceanographic Data Center (NODC)/World Data Center (WDC) in paper as well as electronic form. This product was entitled, Climatological Atlas of the World Ocean. This was followed in 1994 and 1998 with the publications of the World Ocean Atlas 1994, the World Ocean Database 1998, and the World Ocean Atlas 1998. The World Ocean Database 2001 (WOD01) greatly expands on the 1998 product by including data from new instrument types (such as profiling floats), new variables (such as pCO2 and TCO2), and many more historical as well as modern data for the variables in WOD98. WOD01 contains over seven million temperature profiles. The goal in developing and distributing WOD01 is to make available – without restriction – the most complete set of historical ocean profile-plankton measurements possible in electronic form along with appropriate metadata and quality control flags. As with earlier versions of the databases, the data will find use in many different areas of oceanography, meteorology, and climatology. Whether studying the role of the ocean as part of the earth’s climate system, conducting fisheries research, or managing marine resources, scientists and managers depend on observations of the marine environment in order to fulfill their mission. The WOD01 is a product based on data submitted to the NODC and the WDC for Oceanography by individual scientists and scientific teams as well as institutional, national, and regional data centers.
A New University Center in Nuuk, Greenland
Aqqaluk Lynge1, Marianne Stenbaek2
1Inuit Circumpolar Conference, Box 204, Nuuk, D-3900, Greenland, Phone ++299/323632, Fax +299/323001, aqqaluk [at] inuit.org
2Cultural Studies Department, McGill University, 853 Sherbrooke Street, Montreal, QC, H3A 2T6, Canada, Phone 514/398-6579, Fax 514/398-8146, mstenb [at] po-box.mcgill.ca
A new University Center is being established in Nuuk, Greenland. Already many American researchers have been involved in natural and physical sciences research in Greenland. The new University Center will open possibilities of doing social and human sciences research in Greenland. The. poster shows and explains the plans for the Center.
Regional Variation in Cloud Radiative Forcing at the Arctic Surface
Peter J. Minnett1, Tim N. Papakyriakou2, Cristina Ananasso3, Erica L. Key4, Jennifer A. Hanafin5
1Meteorology and Physical Oceanography, University of Miami, RSMAS, 4600 Rickenbacker Causeway, Miami, FL, 33149, USA, Phone 305-361-4104, Fax 305-361-4622, pminnett [at] rsmas.miami.edu
2Geography Department, University of Manitoba, Centre for Earth Observation Science, Winnipeg, MB, Canada
3Institute of Atmospheric Sciences and Climate , Consiglio Nazionale delle Ricerche, Rome, Italy
4Meteorology and Physical Oceanography, University of Miami, Miami, FL, 33149, USA
5Meteorology and Physical Oceanography, University of Miami, Miami, FL, 33149, USA
The effect of the clouds on the radiative fluxes reaching the Earth's surface is an important atmospheric process; and cloud-radiative forcing is a significant factor in the climate system, and the Arctic is an important area to study this process because of its particular sensitivity to climate change. Measurements from a ship and coastal sites in the Canadian Arctic are used to investigate the influence of clouds on the surface radiation budget, and its variation in terms of local characteristics. It is found that cloud radiative forcing is a non-linear function of cloud amount over the low-albedo surface of open water of the North Water Polynya, but a nearly linear function over a high albedo surface of adjacent land-fast ice.
An Ecological Analysis of the Spread of Tuberculosis Among the Inuit of the Canadian Eastern Arctic
Helle Moeller1
1Anthropology, Lakehead University, RR # 13, site 9-34, Thunder Bay, P7B 5E4, Canada, Phone 807-983 2333, helledelle [at] hotmail.com
Many factors influence the disproportionately high tuberculosis incidence in the Canadian Eastern Arctic. Increased knowledge about these factors will improve our ability to tailor appropriate preventative measures, decreasing the incidence of tuberculosis and preventing outbreaks. Some of these factors are systemic, including: a) staff shortage and high turnover rates - both of which compromise surveillance and screening programs, as well the ability to diagnose tuberculosis, b) large reservoirs of infected people who may not have been properly treated in the past, and, c) possible lack of adequate education for health care workers and local residents. Other historical, environmental, bio-cultural, and socio-cultural factors may be important as well.
This poster will synthesize findings from the literature among the latter factors. The analysis draws on the author's experience working with tuberculosis outbreaks in communities of the Canadian Eastern Arctic, which revealed that certain cultural practices probably contributed to the spread of tuberculosis. Intriguing questions for future reseaech are raised.
Biocomplexity in the Arctic: the Bowhead Whale Nexus
Sue Moore1, Carin Ashjian2, Robert Campbell3, Wieslaw Maslowski4, Stephen Okkonen5, Barry Sherr6, Evelyn Sherr7, Yvette Spitz8, Craig George9
1National Marine Mammal Laboratory, National Oceanic and Atmospheric Administration, 7600 Sand Point Way NE, Seattle, WA, 98115, USA, Phone 206-526-4047, Fax 206-526-6615, sue.moore [at] noaa.gov
2Department of Biology, Woods Hole Oceanographic Institution, 266 Woods Hole Road, Woods Hole, MA, 02543, USA, Phone 508-289-3457, Fax 508-457-2134, cashjian [at] whoi.edu
3Graduate School of Oceanography, University of Rhode Island, 50 Lower Campus Road, Kingston, RI, 02881-1901, USA, Phone 401-874-6692, Fax 401-874-6240, campbell [at] gso.uri.edu
4Department of Oceanography, Naval Postgraduate School, 833 Dyer Road Room 331, Monterey, CA, 93943-5122, USA, Phone 831-656-3162, Fax 831-656-2712, maslowsk [at] ncar.ucar.edu
5Institute of Marine Science, University of Alaska Fairbanks, PO Box 1025, Kasilof, AK, AK, 99610, USA, Phone 907-283-3234, Fax 907-283-3234, okkonen [at] alaska.net
6College of Oceanic and Atmospheric Sciences, Oregon State University, 104 Oceanography Admin Building, Corvallis, OR, 97331-5503, USA, Phone 541-737-4369, Fax 541-737-2064, sherrb [at] ucs.orst.edu
7College of Oceanic and Atmospheric Sciences, 104 Ocean Admin Building, Corvallis, OR, 97331-5503, USA, Phone 541-737-4369, Fax 541-737-2064, sherrb [at] ucs.orst.edu
8College of Oceanic and Atmospheric Sciences, Oregon State University, 104 Ocean Admin Building, Corvallis, OR, 97331, USA, Phone 541-737-3227, Fax 541-737-2064, yvette [at] oce.orst.edu
9Department of Wildlife Management, North Slope Borough, PO Box 69, Barrow, AK, 99723, USA, Phone 907-852-0350, Fax 907-852-9848, cgeorge [at] co.north-slope.ak.us
The annual migration of bowhead whales (Balaena mysticetus) past the northern shores of Alaska has provided opportunities for subsistence whaling to the indigenous peoples of this region for generations. Subsistence whaling remains an integral part of a mixed hunting/wage economy of this region and a key component to maintenance of traditional lifestyles. During the fall migration, bowheads often aggregate to feed on zooplankton concentrations near Kaktovik and Barrow, Alaska and are accessible to hunters from these communities. Bowhead whale distribution, relative abundance and availability to the Native subsistence hunters likely are influenced by a complex suite of factors including: near-term oceanographic variability resulting from local (i.e. weather) and basin-scale shifts in atmospheric forcing; longer-term global climate fluctuations; and localized human activity such as that associated with oil and gas exploration and production. The subsistence whaling tradition and way of life are particularly vulnerable to changes in resource availability in response both to environmental changes and human generated pressure.
Numerical modeling of atmospheric and oceanographic conditions during the past 50 years has identified two major climatic regimes for the Arctic Ocean that embody many of the variations in climate, weather, and oceanographic circulation observed in the region. These regimes likely have dramatic impacts on the regional ecosystems, with cascading effects through the food chain to bowhead whales. This complex suite of human-environment-whale interactions comprises a biocomplex system of coupled natural and human interactions that is vulnerable both to natural and human generated change. We propose* to quantify linkages in this system to understand how physical-biological and human-natural coupling in the Western Arctic Ocean influences bowhead whale behavioral ecology and Native subsistence harvests. Interactions within the natural-human system will be quantified by combining retrospective analyses with biological-physical modeling of the atmospher-ice-ocean system and the climatic variability therein. The goal is to identify mechanisms that produce bowhead whale prey aggregations thereby influencing whale distributions and hunting success. Local monitoring (i.e. citizen science) of oceanographic conditions will be conducted to verify model predictions during the period of the study, while collaboration with investigators that target critical components of the system, or which expand upon the local sampling program, will provide a broader scope of information. The proposed fusion of biological and physical models is a new approach and will lead to an improved understanding of the bio-physical linkages involved in the cascading effects of climate variability in the Arctic Ocean ecosystem, and to better definition of the critical terms in the coupled human-natural system. The proposed work contains also a strong outreach and educational component involving the communities of Barrow and Kaktovik and will incorporate traditional knowledge and local records regarding bowhead whale distribution, bowhead whale feeding behavior, and oceanographic conditions in developing the sampling program and in verifying retrospective modeling predictions.
....................................................................
* Abstract from Project Summary: NSF Proposal No. 0216045
Climate and Subsistence Hunting: The Sustainability of Arctic Communities
Craig Nicolson1, Jack Kruse2, Gary Kofinas3, Matthew D. Berman4, Don Russell5, Brad Griffith6, Craig George7, Harry Brower, Jr.8, Stephen Braund9
1Department of Natural Resources Conservation, University of Massachusetts, 160 Holdsworth Way, Amherst, MA, 01003, USA, Phone 413/545-3154, Fax 413/545-4358, craign [at] forwild.umass.edu
2Department of Geosciences, University of Massachusetts, Leverett, MA, USA, afjak [at] uaa.alaska.edu
3Institute of Arctic Studies, Dartmouth College, PO Box 832, Wilson, WY, 83014, USA, gary.kofinas [at] dartmouth.edu
4Institute of Social and Economic Research, University of Alaska Anchorage, 3211 Providence Drive, Anchorage, AK, 99508, USA, auiser [at] uaa.alaska.edu
5Environment Canada, Canadian Wildlife Service, 91782 Alaska Highway, Whitehorse, YT, Y1A 5B7, Canada, don.russell [at] ec.gc.ca
6Alaska Cooperative Fish and Wildlife Research Unit, University of Alaska Fairbanks, PO Box 757020, Fairbanks, AK, 99775, USA, ffdbg [at] uaf.edu
7Department of Wildlife Management, North Slope Borough, PO Box 69, Barrow, AK, 99723, USA, cgeorge [at] co.north-slope.ak.us
8Department of Wildlife Management, North Slope Borough, PO Box 69, Barrow, AK, 99723, USA
9Stephen Braund and Associates, PO Box 101480, Anchorage, AK, 99510, USA, srba [at] alaska.net
The arctic climate system is marked by substantial inter-annual variability, and it determines many of the environmental conditions that influence the distribution of migratory subsistence resources such as bowhead whale and caribou. Will the indigenous communities of the arctic successfully continue to harvest these resources in the face of a changing climate?
We present two case studies: spring bowhead whale hunting in Barrow, Alaska, and caribou hunting in Old Crow, Canada. In both cases, we present an analytical framework for understanding and quantifying the interactions between the natural and human systems in order to understand the influence of the climate system on local hunting effort and success.
In this presentation we summarize model relationships and data sources, describing in particular how local knowledge contributed to building relationships and assumptions. We then outline how we used a simulation model to explore the interactions between these relationships and to assess how a warmer climate might affect the local subsistence economy and the well-being of residents. Our results suggest that local policies as well as sharing of hunting gear and caribou harvests help ensure that most people are able to meet their subsistence needs despite varying levels of wage employment and money income.
Biocomplexity of Marine and Terrestrial Environments and Human Populations in Iceland
Astrid Ogilvie1
1Institute of Arctic & Alpine Research, University of Colorado at Boulder, 1560 30th Street, Campus Box 450, Boulder, CO, 80303, USA, Phone 303-492-6072, Fax 303-492-6388, ogilvie [at] spot.colorado.edu
The research described in this talk may be seen in the context of current concerns regarding potential future global and Arctic changes, the crisis in the world's fisheries, and issues regarding land-use and continuing erosion of land surfaces in Iceland. Possible future climate and environmental changes may radically affect the lives of those living in the Arctic. The consequences of such changes cannot be predicted and understood if there is no knowledge and understanding of the past and present. This talk will focus on present and historical dynamics of linkages and interactions between human populations and the biological and physical environment of Iceland. Both terrestrial and marine components will be considered. From the time of the earliest settlers to the late-nineteenth century, the most important economic activity in Iceland has been farming. Because of the unsuitability of the climate for extensive grain-growing, this was based on animal husbandry. The most important livestock animals in Iceland have been sheep, cattle and horses, and the most important crop has been the grass and hay on which these animals depended for food. In the past, lack of food for the livestock could ultimately lead to deaths from hunger, and malnutrition-related diseases among humans. Land-use activity, in particular sheep grazing, has been a major contributory factor to soil erosion, a serious concern in Iceland. The rate of erosion increased greatly through the eighteenth century and reached a maximum during the nineteenth and early twentieth centuries. During this period, numerous farms were abandoned, especially in southern and northeastern Iceland. Fishing developed rapidly as a major industry in the nineteenth century. Early in the twentieth century, fishers and boat owners gradually replaced the landed elite as the economy shifted from a somewhat stagnant agriculture to expansive fishing. The twentieth century has seen great changes in fisheries catches, partly at least as a consequence of environmental changes. Although Iceland as a whole is considered here, two specific locations are analyzed more closely. These are the Myvatn area in the northeast of Iceland, a community based primarily on land-use, and the Vestmannaeyjar (Westman Islands) located off the south of Iceland, mainly a fishing community. Developments and adaptive strategies in these communities are considered in the context of: climate variations in Iceland during the twentieth century and earlier; records of hay yield and farm stocking strategies; and fisheries records. Although the research described in this presentation will focus on the present and immediate past, possible future changes are also considered.
Quantifying Pan-Arctic Environmental Change
James E. Overland1
1Pacific Marine Environmental Lab./NOAA, 7600 Sand Point Way NE, Seattle, WA, 98115, USA, Phone 206.526.6795, Fax 206.526.6795, overland [at] pmel.noaa.gov
The Arctic has undergone major temperature swings over the last 100 years. Over the past three decades, demonstrable pan-Arctic changes have occurred in many components of the physical and biological system. The areal coverage of sea ice has diminished, and sea level pressures in the central Arctic have decreased, resulting in a shift of wind and heat flux patterns. Warmer surface temperatures are observed in northern Europe during winter and Alaska and northwest Canada during spring, there is an increase in the frequency of years with cold temperature anomalies in the lower stratosphere over high latitudes, and permafrost temperatures have risen in Siberia and Alaska with increased erosion. Satellite estimates of "greening" have increased over both the eastern and western hemispheres, with longer growing seasons and changes in the character of the tundra. The influence of warm Atlantic water in the Arctic Ocean is becoming more widespread and intense, with implications for the stability of the water column. These changes are robust, and many other biological and physical changes are suggested: increases in cod in the Barents and shrimp off of southern Greenland, increases in calf survival for some caribou populations in North America, and declines and redistributions of marine mammal populations, although causes for these changes are less certain.
Extrapolation of atmospheric and sea ice records from the last 100 years implies a reversal of present trends in 2000–2030, while IPCC climate models imply a continuation of the current course. At present, neither evidence nor understanding is available to unequivocally distinguish between these scenarios. A promising method for future detection of change is to use a multivariate approach. Biological and terrestrial variables are often better indicators of decadal change than physical variables because they integrate over the large meteorological and oceanographic interannual and intraseasonal variability. A data collection for 1965 – 1995 of 85 variables representing 7 data types shows broad pan-Arctic covariability for a shift in ecosystems near 1989. Understanding biocomplexity is an important factor in developing an Arctic change detection system as extremes in the physical environment lasting one or two years can precipitate biological/ecosystem changes that last several decades.
Quantifying Pan-Arctic Environmental Change
James E. Overland1
1Pacific Marine Environmental Lab./NOAA, 7600 Sand Point Way NE, Seattle, WA, 98115, USA, Phone 206.526.6795, Fax 206.526.6795, overland [at] pmel.noaa.gov
The Arctic has undergone major temperature swings over the last 100 years. Over the past three decades, demonstrable pan-Arctic changes have occurred in many components of the physical and biological system. The areal coverage of sea ice has diminished, and sea level pressures in the central Arctic have decreased, resulting in a shift of wind and heat flux patterns. Warmer surface temperatures are observed in northern Europe during winter and Alaska and northwest Canada during spring, there is an increase in the frequency of years with cold temperature anomalies in the lower stratosphere over high latitudes, and permafrost temperatures have risen in Siberia and Alaska with increased erosion. Satellite estimates of "greening" have increased over both the eastern and western hemispheres, with longer growing seasons and changes in the character of the tundra. The influence of warm Atlantic water in the Arctic Ocean is becoming more widespread and intense, with implications for the stability of the water column. These changes are robust, and many other biological and physical changes are suggested: increases in cod in the Barents and shrimp off of southern Greenland, increases in calf survival for some caribou populations in North America, and declines and redistributions of marine mammal populations, although causes for these changes are less certain.
Extrapolation of atmospheric and sea ice records from the last 100 years implies a reversal of present trends in 2000–2030, while IPCC climate models imply a continuation of the current course. At present, neither evidence nor understanding is available to unequivocally distinguish between these scenarios. A promising method for future detection of change is to use a multivariate approach. Biological and terrestrial variables are often better indicators of decadal change than physical variables because they integrate over the large meteorological and oceanographic interannual and intraseasonal variability. A data collection for 1965 – 1995 of 85 variables representing 7 data types shows broad pan-Arctic covariability for a shift in ecosystems near 1989. Understanding biocomplexity is an important factor in developing an Arctic change detection system as extremes in the physical environment lasting one or two years can precipitate biological/ecosystem changes that last several decades.
Interactions between hydrological and biotic process in the Arctic landscape
Joshua Schimel1
1Ecology, Evolution, & Marine Biology & Environmental Studies, University of California, Santa Barbara, CA, 93106, USA, Phone 805/893-7688, Fax 805/893-4724, Schimel [at] lifesci.lscf.ucsb.edu
Traditionally, terrestrial ecology had focused on individual study plots in distinct community types, in which the physical system was largely seen as a driver of biotic activity. This approach, however, has changed dramatically. The tundra is no longer a collection of community types, but an integrated landscape in which physical and biotic systems strongly feed back on each other. In this view, freshwater is a critical integrating force, moving carbon and nutrients across the landscape and into streams, lakes, and ultimately the ocean. One important aspect of this development is an appreciation of winter. We are coming to better understand how winter processes (snow timing and distribution) drive not only hydrology but also the biotic systems, which we used to assume "turn off" during the winter. Strong feedbacks develop through the winter, for example, shrubs trap blowing snow, producing a better insulating layer. Warmer, though still frozen, soils mineralize nitrogen that is available for plant uptake in the spring (or loss at snowmelt). Higher nutrient availability in turn encourages shrub growth, closing a positive feedback loop that has the potential to drastically change the tundra landscape. Such feedbacks may develop on a local scale, but apply broadly across the Arctic. Understanding the functioning of these feedback mechanisms has led to major rethinking in our understanding of the Arctic system.
Biocomplexity of Frost Boil Ecosystem on the Arctic Slope, Alaska
Donald A. Walker1, Vladimir E. Romanovsky2, William B. Krantz3, Chien L. Ping4, Rorik A. Peterson 5, Martha K. Raynolds6, Howard E. Epstein7, Jiong G. Jia8, David C. Wirth9
1Institute of Arctic Biology, University of Alaska, PO Box 757320, Fairbanks, AK, 99775, USA, Phone 907-474-2460, Fax 907-474-7666, ffdaw [at] uaf.edu
2Geophysical Institute, University of Alaska, PO Box 757320, Fairbanks, AK, 99775, USA, Phone 907-474-7459, Fax 907-474-7290, ffver [at] uaf.edu
3Institute of Arctic and Alpine Research (INSTAAR), University of Colorado, Campus Box 450, Boulder, CO, 80309, USA, Phone 303-492-7517, Fax 303-492-4637, krantz [at] spot.colorado.edu
4Department of Plant, Animal, and Soil Sciences, Palmer Research Center, 533 E Fireweed Avenue, Palmer, AK, USA, Phone 907-746-9462, Fax 907-746-2677, pfclp [at] uaa.alaska.edu
5USA
6Institute of Arctic Biology, University of Alaska Fairbanks, PO Box 757000, Fairbanks, AK, 99775, USA, Phone 907-474-2459, fnmkr [at] uaf.edu
7Department of Environmental Sciences, University of Virginia, PO Box 400123, Charlottesville, VA, 22904, USA, Phone 434-924-4308, Fax 434-982-2137, hee2b [at] virginia.edu
8Department of Environmental Sciences, University of Virginia, PO Box 400123, Charlottesville, VA, 22904, USA, Phone 434-982-2337, Fax 434-982-2137, jj4u [at] virginia.edu
9USA
The central goal of this project is to understand the complex linkages between biogeochemical cycles, vegetation, disturbance, and climate across the full summer temperature gradient in the Arctic in order to better predict ecosystem responses to changing climate. We focus on frost-boils because: (1) The processes that are involved in the self-organization of these landforms drive biogeochemical cycling and vegetation succession of extensive arctic ecosystems. (2) These ecosystems contain perhaps the most diverse and ecologically important zonal ecosystems in the Arctic and are important to global carbon budgets. (3) The complex ecological relationships between patterned-ground formation, biogeochemical cycles, and vegetation and the significance of these relationships at multiple scales have not been studied. (4) The responses of the system to changes in temperature are likely to be nonlinear, but can be understood and modeled by examining the relative strengths of feedbacks between the components of the system at several sites along the natural arctic temperature gradient.
We propose to examine disturbed and undisturbed patches associated with frost-boil ecosystems, from polar deserts of northern Canada to shrub tundra systems in Alaska. Frost boils are caused by soil heave in small, regularly spaced, circular highly disturbed patches, 1-3 m in diameter. The processes of frost-boil formation is currently poorly understood, so full knowledge of the biogeochemical system first requires a better understanding of the process of frost heave itself and how it is modified by interactions with climate and vegetation. A recent Differential-Frost-Heave (DFH) model by Peterson and Krantz provides considerable insight to the self-organization processes in frost boils. This physically based model can predict many phenomena associated with frost boils including the amount of heave, spacing and size of the boils. Early model results suggest that the ground-surface temperature is a primary control of frost-boil formation. Vegetation and/or snow cover can constrain the development of frost boils by insulating the soils. Climate and the level of disturbance caused by frost heave strongly influence the rate of organic-matter accumulation and biogeochemical cycles within the tundra soils. We will examine the full implications of this interaction by studying how frost heave affects nitrogen and carbon pools and rates of nitrogen mineralization along the climate gradient. We propose to answer six major questions related to the biocomplexity of frost-boil ecosystems:
(1) How does the self-organization associated with frost boils occur?
(2) How does the frost heave affect the soil biogeochemical processes within and between the frost boils?
(3) How do frost heave and biogeochemical processes affect plant communities?
(4) How do the biological processes in turn feed back to control frost heave?
(5) How do interactions between biogeochemistry, cryotrubation and vegetation change along the existing arctic climate gradient?
(6) How do the complex patterns associated with frost boils affect the tundra systems in a hierarchy of spatial-temporal scales?
The response of the system to summer temperature is nonlinear. We propose to dissolve the complex system in into linear models by examining the relationship between frost heave, carbon, nitrogen, and vegetation at a series of sites within five bioclimatic subzones along the temperature gradient. We will use ordination, path analysis, and information theory to examine the complex multivariate relationships between biogeochemistry, cryotrubation, vegetation, and the environment. We hypothesize that zonal sites in areas with intermediate summer warmth should have the highest measures of several key ecosystem functions (e.g., mineralization rates, nitrogen pools, biodiversity). In years 1 and 2, the field studies will focus along the Dalton Highway in northern Alaska. This region is well known and provides an exceptional opportunity because it has a particularly sharp boundary that demarcates essentially High-Arctic soils with abundant frost boils from Low Arctic soils with few frost boils. Extensive ecosystem, snow, and ground-temperature data are available from several sites in this region. In years 2, 3 and 4, we will extend the research into more remote areas in the Canadian Arctic where there are colder bioclimate subzones not represented in northern Alaska.
To arrive at a predictive capability for biogeochemical response and plant community formation, we will use output from the DFH model to help parameterize a vegetation-change model (ArcVeg). The project has four research components: (1) Frost Boil Dynamics, Climate, and Permaforst, (2) Soil and Biogeochemistry, (3) Vegetation, and (4) Modeling Nutrient and Vegetation Dynamics. We also propose a major educational component of the study. Students, as part of Dr. Bill Gould’s Arctic Field Ecology course, will interact with the scientists. They will be actively engaged in the research activities as part of expeditions that will allow them to study biocomplexity in the Arctic. We are also proposing to host an arctic biocomplexity workshop early in the project and a synthesis workshop in Year 4.
Biocomplexity of Frost Boil Ecosystem on the Arctic Slope, Alaska
Donald A. Walker1, Vladimir E. Romanovsky2, William B. Krantz3, Chien L. Ping4, Rorik A. Peterson 5, Martha K. Raynolds6, Howard E. Epstein7, Jiong G. Jia8, David C. Wirth9
1Institute of Arctic Biology, University of Alaska, PO Box 757320, Fairbanks, AK, 99775, USA, Phone 907-474-2460, Fax 907-474-7666, ffdaw [at] uaf.edu
2Geophysical Institute, University of Alaska, PO Box 757320, Fairbanks, AK, 99775, USA, Phone 907-474-7459, Fax 907-474-7290, ffver [at] uaf.edu
3Institute of Arctic and Alpine Research (INSTAAR), University of Colorado, Campus Box 450, Boulder, CO, 80309, USA, Phone 303-492-7517, Fax 303-492-4637, krantz [at] spot.colorado.edu
4Department of Plant, Animal, and Soil Sciences, Palmer Research Center, 533 E Fireweed Avenue, Palmer, AK, USA, Phone 907-746-9462, Fax 907-746-2677, pfclp [at] uaa.alaska.edu
5USA
6Institute of Arctic Biology, University of Alaska Fairbanks, PO Box 757000, Fairbanks, AK, 99775, USA, Phone 907-474-2459, fnmkr [at] uaf.edu
7Department of Environmental Sciences, University of Virginia, PO Box 400123, Charlottesville, VA, 22904, USA, Phone 434-924-4308, Fax 434-982-2137, hee2b [at] virginia.edu
8Department of Environmental Sciences, University of Virginia, PO Box 400123, Charlottesville, VA, 22904, USA, Phone 434-982-2337, Fax 434-982-2137, jj4u [at] virginia.edu
9USA
The central goal of this project is to understand the complex linkages between biogeochemical cycles, vegetation, disturbance, and climate across the full summer temperature gradient in the Arctic in order to better predict ecosystem responses to changing climate. We focus on frost-boils because: (1) The processes that are involved in the self-organization of these landforms drive biogeochemical cycling and vegetation succession of extensive arctic ecosystems. (2) These ecosystems contain perhaps the most diverse and ecologically important zonal ecosystems in the Arctic and are important to global carbon budgets. (3) The complex ecological relationships between patterned-ground formation, biogeochemical cycles, and vegetation and the significance of these relationships at multiple scales have not been studied. (4) The responses of the system to changes in temperature are likely to be nonlinear, but can be understood and modeled by examining the relative strengths of feedbacks between the components of the system at several sites along the natural arctic temperature gradient.
We propose to examine disturbed and undisturbed patches associated with frost-boil ecosystems, from polar deserts of northern Canada to shrub tundra systems in Alaska. Frost boils are caused by soil heave in small, regularly spaced, circular highly disturbed patches, 1-3 m in diameter. The processes of frost-boil formation is currently poorly understood, so full knowledge of the biogeochemical system first requires a better understanding of the process of frost heave itself and how it is modified by interactions with climate and vegetation. A recent Differential-Frost-Heave (DFH) model by Peterson and Krantz provides considerable insight to the self-organization processes in frost boils. This physically based model can predict many phenomena associated with frost boils including the amount of heave, spacing and size of the boils. Early model results suggest that the ground-surface temperature is a primary control of frost-boil formation. Vegetation and/or snow cover can constrain the development of frost boils by insulating the soils. Climate and the level of disturbance caused by frost heave strongly influence the rate of organic-matter accumulation and biogeochemical cycles within the tundra soils. We will examine the full implications of this interaction by studying how frost heave affects nitrogen and carbon pools and rates of nitrogen mineralization along the climate gradient. We propose to answer six major questions related to the biocomplexity of frost-boil ecosystems:
(1) How does the self-organization associated with frost boils occur?
(2) How does the frost heave affect the soil biogeochemical processes within and between the frost boils?
(3) How do frost heave and biogeochemical processes affect plant communities?
(4) How do the biological processes in turn feed back to control frost heave?
(5) How do interactions between biogeochemistry, cryotrubation and vegetation change along the existing arctic climate gradient?
(6) How do the complex patterns associated with frost boils affect the tundra systems in a hierarchy of spatial-temporal scales?
The response of the system to summer temperature is nonlinear. We propose to dissolve the complex system in into linear models by examining the relationship between frost heave, carbon, nitrogen, and vegetation at a series of sites within five bioclimatic subzones along the temperature gradient. We will use ordination, path analysis, and information theory to examine the complex multivariate relationships between biogeochemistry, cryotrubation, vegetation, and the environment. We hypothesize that zonal sites in areas with intermediate summer warmth should have the highest measures of several key ecosystem functions (e.g., mineralization rates, nitrogen pools, biodiversity). In years 1 and 2, the field studies will focus along the Dalton Highway in northern Alaska. This region is well known and provides an exceptional opportunity because it has a particularly sharp boundary that demarcates essentially High-Arctic soils with abundant frost boils from Low Arctic soils with few frost boils. Extensive ecosystem, snow, and ground-temperature data are available from several sites in this region. In years 2, 3 and 4, we will extend the research into more remote areas in the Canadian Arctic where there are colder bioclimate subzones not represented in northern Alaska.
To arrive at a predictive capability for biogeochemical response and plant community formation, we will use output from the DFH model to help parameterize a vegetation-change model (ArcVeg). The project has four research components: (1) Frost Boil Dynamics, Climate, and Permaforst, (2) Soil and Biogeochemistry, (3) Vegetation, and (4) Modeling Nutrient and Vegetation Dynamics. We also propose a major educational component of the study. Students, as part of Dr. Bill Gould’s Arctic Field Ecology course, will interact with the scientists. They will be actively engaged in the research activities as part of expeditions that will allow them to study biocomplexity in the Arctic. We are also proposing to host an arctic biocomplexity workshop early in the project and a synthesis workshop in Year 4.